OPERA was specifically designed to identify the τ via the topological observation of its decay, followed by a kinematical analysis of the event. This is done by means of a hybrid apparatus that combines real-time detection techniques (electronic detectors) and the Emulsion Cloud Chamber (ECC) technique. An ECC detector is made of passive material plates, used as target, alternated with nuclear emulsion films used as tracking devices with sub-micrometric accuracy.
The ECC technique has a long history, being adopted soon after the Second World War in experiments on cosmic-rays and leading to the first observation of charmed particles in 1971, three years before its observation in accelerator experiments. At that time, an emulsion experiment was almost entirely based on the visual inspection of the films by experienced operators and it was close to be abandoned until the development of fully
automated microscope scanning systems brought this detector to a revival in the early nineties. The DONUT experiment, which has first observed the ντ is a successful application of the ECC technology at a mass scale of 100 kg. The sub-micrometric position accuracy and the use of passive material allow for momentum measurement of charged particles by the detection of multiple Coulomb scattering, identification and measurement of electromagnetic showers and electron/pion separation. Such studies have been conducted during the last years in the framework of the OPERA experiment. The ECC has proven the unique feature of combining a tracker with an extremely high accuracy and the capability of performing kinematical variable measurements.
Today OPERA brings the ECC technology to a much larger scale: the basic unit of the experiment is a "brick" made of 56 plates of lead (1 mm thick) interleaved with nuclear emulsion films (industrially produced) for a total weight of 8.3 kg. 150000 of such target units have been assembled to reach an overall mass of 1.25 kton. The bricks are arranged in 62 vertical "walls", transverse to the beam direction, interleaved with planes of plastic scintillators. The detector is subdivided in two identical units (supermodules), each consisting of 31 walls and 31 double layers of scintillator planes followed by a magnetic spectrometer (see Fig.1).
The electronic detectors have the task to trigger the data acquisition, identify and measure the trajectory of charged particles and locate the brick where the interaction occurred. The momentum of muons is measured by the spectrometers while their trajectories are traced back through the scintillator planes up to the brick where the track originates. When no muons are observed, the scintillator signals produced by electrons or hadronic showers are used to predict the location of the brick that contains the primary neutrino interaction vertex, hence providing "time resolution" to the emulsions.
The selected brick is then extracted from the target and the two interface emulsion films, called "Changeable Sheets" (CS), attached on the downstream face of the brick are developed. If tracks related to the neutrino interaction are observed in these CS, the films of the brick are also developed and the tracks are followed back by fully automated scanning microscopes until the vertex is located. The analysis of the event topology at the primary vertex will then select possible τ candidates. The latter might include one track that shows a clear "kink" due to the decay-in-flight of the tau (long decays) or an anomalous impact parameter with respect to the primary vertex (short decays) compatible with a decay-in-flight in the first lead plate. They will then be confirmed by a kinematical analysis at the primary and decay vertices. The modular structure of the target allows to extract only the bricks actually hit by the neutrinos, thus achieving a quasi online analysis of the interaction and minimizing the target mass reduction during the run.
The structure of the OPERA detector is illustrated in Figs.1 and 2. Each brick wall consisting of 3328 bricks supported by a light stainless steel structure is followed by a double layer of plastic scintillators (Target Trackers, TT) that provide real-time detection of the outgoing charged particles. The instrumented target is followed by a magnetic spectrometer consisting of a large iron magnet instrumented with plastic Resistive Plate Chambers (RPC). The deflection of charged particles inside the magnetized iron is measured by six stations of drift tubes (Precision Trackers, PT). Left-right ambiguities in the reconstruction of particle trajectories inside the PT are removed by means of additional RPC (XPC) with readout strips rotated by 45 degrees with respect to the horizontal and positioned near the first two PT stations. An instrumented target and its spectrometer is called a “supermodule“ (SM). The whole OPERA detector is made of two such supermodules. Finally, two glass RPC planes mounted in front of the first target (VETO) allow to reject charged particles originating from outside the target fiducial region, coming from neutrino interactions in the surrounding rock material and in the Borexino experiment.
The installation of OPERA in the Hall C of LNGS started in Summer 2003 with the positioning of the two lower return yokes of the magnets. After completion of the first magnet a support structure was installed in August 2004 to hold the first instrumented target. It consists of support rails for the walls, a top platform housing detector electronics and a seismic damping structure. The installation of the walls started in October 2004 and was completed in May 2006. At the time of the first CNGS pilot run (Summer 2006) all electronic detectors except the PT planes of SM2 and the VETO were operational. The PT and VETO installation was completed in 2007. Each wall and tracker plane has a surface of about 6.7x6.7 m2, transverse to the beam direction. The overall dimension of the detector is 10x10x20 m3 for a total weight of about 4 kton.
During the mounting of the electronic detectors, emulsion films coming from Japan were progressively stored in a dedicated bunker in the LNGS Hall B. Massive brick production in the underground hall (to minimize the collection of cosmic-ray tracks) started after the installation of the Brick Manipulator System (BMS) and of the Brick Assembly Machine (BAM). Bricks produced by the BAM were stored in a cylindrical container (a "drum" containing 234 bricks). Thereafter, drums were transported to Hall C and were positioned on the loading station using a crane. The BMS robot extracted the bricks from the drum and inserted them into the walls. Brick mass production started in Spring 2007 and, for the 2008 data taking period, 97% of the ECC bricks have been produced and loaded to their final position. OPERA is the first very large scale emulsion experiment. Just to give an idea, the 150000 ECC bricks include about 110,000 m2 emulsion films and 105,000 m2 lead plates. The scanning of the events is performed with more than 30 fully automated microscopes, each of them faster by about two orders of magnitude than those used e.g. in the CHORUS experiment.
OPERA is the first very large scale emulsion experiment. Just to give an idea, the 150000 ECC bricks include about 110,000 m2 emulsion films and 105,000 m2 lead plates. The scanning of the events is performed with more than 30 fully automated microscopes, each of them faster by about two orders of magnitude than those used e.g. in the CHORUS experiment.
OPERA is currently in data taking. In particular, in 2008 the CNGS beam delivered 1.78 x 10
19 protons on target. Corresponding to this statistics OPERA recorded about 10100 events correlated in time with the CNGS beam, among which 1700 candidates of neutrino interactions in the bricks.
Figure 1 - Fish-eye side view of the OPERA detector. The upper red horizontal lines indicate the position of the two identical supermodules (SM1 and SM2). The "target area" is made of walls filled with ECC bricks interleaved with planes of plastic scintillators (TT): the black covers are the end-caps of the TT. Arrows also show the position of the VETO planes, the drift tubes (PT) surrounded by the XPC, the magnets and the RPC installed between the magnet iron slabs. The Brick Manipulator System (BMS) is also visible.
|
Figure 2 - Artistic view of the OPERA detector. The yellow arrow in front of the VETO indicates the direction of the incoming CNGS neutrino beam.
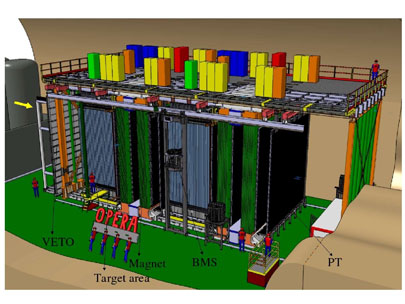 |