The goal of CRESST is the direct detection of elementary particle dark matter and the elucidation of its nature. CRESST is presently one of the most advanced deep underground, low background, cryogenic facilities. A more general description of CRESST can be found here. The search for dark matter and the understanding of its nature remains one of the central and most fascinating problems of our time in physics, astronomy and cosmology. There is strong evidence for it on all scales ranging from dwarf galaxies, through spiral galaxies like our own, to large scale structures. The history of the universe is difficult to reconstruct without it, be it big bang nucleosynthesis [1] or the formation of structure [2]. Probably the strongest evidence that dark matter is still present nowadays comes from the observation of the bullet cluster collision [3].
The detectors developed by the CRESST collaboration consist of a dielectric crystal with a small superconducting W-film deposited on the surface. The detectors are run near 15 mK, in the middle of the W-film transition between its superconducting and normal states. Here a small change in the temperature of the superconducting film leads to a relatively large change in its resistance that we measure with a SQUID. To a good approximation, the high frequency phonons, created by a particle interaction or the absorption of light do not thermalize in the crystal until they are absorbed in the superconducting film. The energy resolution is then only moderately dependent on the mass of the crystal, so that
large detectors can be realised. The high sensitivity of our thermometers enables us to measure the light emitted from a scintillating crystal with a small detector of the same type.
In the first stage of CRESST we were using sapphire detectors with a mass of 262g and an energy threshold of 500 eV. These detectors are most sensitive to low mass WIMPs with spin dependent interaction. Results are published in [4].
The passive techniques of background reduction - the employment of radiopure materials and a low background environment - is imperative for every dark matter search. The still remaining background rate is dominated by electrons and photons from radioactive contaminations, which exclusively produce electron recoils in the detector. In contrast, WIMPs as well as neutrons scatter elastically off nuclei. Therefore, dramatic improvements in sensitivity are to be expected if the detector itself is capable of distinguishing electron from nuclear recoils.
For the second phase of the experiment, CRESST II, we have developed calorimeters, presently using CaWO4 crystals, with simultaneous measurement of scintillation light and phonons. We found that these devices can discriminate clearly nuclear recoils from electron recoils. As shown in Fig. 1, this allows the rejection of radioactive background caused by photons and electrons with an efficiency of better than 99% for nuclear recoil energies above 15 keV. The mass of a single detector module is about 300g. With a total number of 33 modules CRESST II will have a mass of about 10 kg.
For the short term we plan to continue our current measurements with detectors made from CaWO4, which are sensitive to medium and high mass WIMPs. The detector mass will be increased to reach a better sensitivity, but there is also the possibility for building in the near future detectors which consist of other materials like ZnWO4 and test in this way the properties of an eventually discovered new particle.
[1] J. Audouze, Nucl. Phys. News 8 (1998) No.2, 22
[2] S. Dodelson, E.I. Gates, M.S. Turner, Science 274 (1996) 69
[3] Douglas Clowe et al., A direct empirical proof of the existence of dark matter,
Astrophys.J. 648:L109-L113, 2006 and arXiv:astro-ph/0608407
[4] G. Angloher et al., Astroparticle Physics 18 (2002) 43-55.
[5] G. Angloher et al., Astroparticle Physics, Commissioning Run of the CRESST-II Dark Matter Search, http://dx.doi.org/10.1016/j.astropartphys.2009.02.007
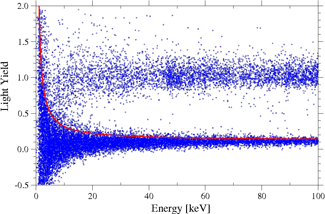
Fig. 1: Discrimination of electron and nuclear recoils in CaWO4 by the simultaneous detection of phonons and scintillation photons. Nuclear recoils produced by neutrons or WIMPs produce less scintillation light than an electron recoil (produced by electrons or photons) of the same energy. Plotting pulse height in the light detector (normalized to electromagnetic interaction) versus pulse height in the phonon detector, two well separated bands can be seen. The upper one is caused by electron recoils and the lower one by nuclear recoils from a neutron calibration. Below the red line 90% of the neutrons are expected. (From [5])
Fig. 2: Low-energy event distribution measured with a 300 g CaWO4 detector module during the commissioning run. The axes are the same as in Fig. 1. Below the dashed black curve 90% of all nuclear recoils, and below the solid red curve 90% of the tungsten recoils are expected.
Fig. 3: WIMP-nucleon cross section sensitivities (90% CL) for scalar interactions as a function of the WIMP mass. The data from the commissioning run of CRESST in 2007 are compared with other leading experiments. (From [5])
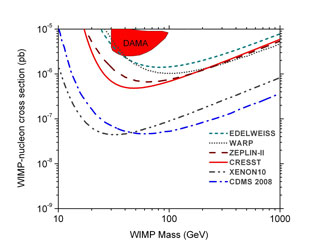